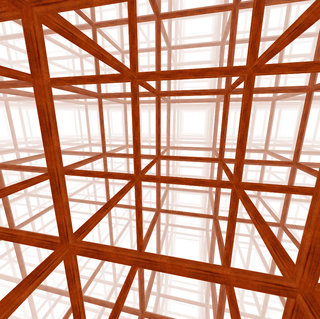
In geometry, a honeycomb is a space filling or close packing of polyhedral or higher-dimensional cells, so that there are no gaps. It is an example of the more general mathematical tiling or tessellation in any number of dimensions. Its dimension can be clarified as n-honeycomb for a honeycomb of n-dimensional space.

In four-dimensional Euclidean geometry, the 24-cell honeycomb, or icositetrachoric honeycomb is a regular space-filling tessellation of 4-dimensional Euclidean space by regular 24-cells. It can be represented by Schläfli symbol {3,4,3,3}.

In geometry, the alternated hypercube honeycomb is a dimensional infinite series of honeycombs, based on the hypercube honeycomb with an alternation operation. It is given a Schläfli symbol h{4,3...3,4} representing the regular form with half the vertices removed and containing the symmetry of Coxeter group for n ≥ 4. A lower symmetry form can be created by removing another mirror on an order-4 peak.
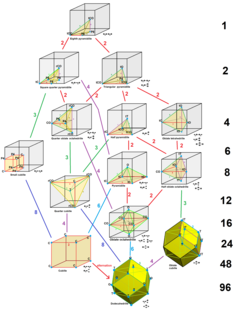
In geometry, John Horton Conway defines architectonic and catoptric tessellations as the uniform tessellations of Euclidean 3-space and their duals, as three-dimensional analogue of the Platonic, Archimedean, and Catalan tiling of the plane. The singular vertex figure of an architectonic tessellation is the dual of the cell of catoptric tessellation. The cubille is the only Platonic (regular) tessellation of 3-space, and is self-dual. There are other uniform honeycombs constructed as prismatic stacks which are excluded from these categories.

In four-dimensional Euclidean geometry, the birectified 16-cell honeycomb is a uniform space-filling tessellation in Euclidean 4-space.

The bitruncated cubic honeycomb is a space-filling tessellation in Euclidean 3-space made up of truncated octahedra. It has 4 truncated octahedra around each vertex. Being composed entirely of truncated octahedra, it is cell-transitive. It is also edge-transitive, with 2 hexagons and one square on each edge, and vertex-transitive. It is one of 28 uniform honeycombs.

In geometry, a convex uniform honeycomb is a uniform tessellation which fills three-dimensional Euclidean space with non-overlapping convex uniform polyhedral cells.
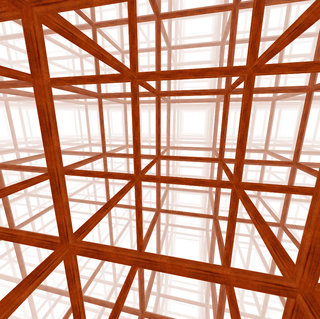
The cubic honeycomb or cubic cellulation is the only proper regular space-filling tessellation in Euclidean 3-space, made up of cubic cells. It has 4 cubes around every edge, and 8 cubes around each vertex. Its vertex figure is a regular octahedron. It is a self-dual tessellation with Schläfli symbol {4,3,4}. John Horton Conway calls this honeycomb a cubille.

In five-dimensional Euclidean geometry, the cyclotruncated 5-simplex honeycomb or cyclotruncated hexateric honeycomb is a space-filling tessellation. It is composed of 5-simplex, truncated 5-simplex, and bitruncated 5-simplex facets in a ratio of 1:1:1.

In geometry, the cyclotruncated simplectic honeycomb is a dimensional infinite series of honeycombs, based on the symmetry of the affine Coxeter group. It is given a Schläfli symbol t0,1{3[n+1]}, and is represented by a Coxeter-Dynkin diagram as a cyclic graph of n+1 nodes with two adjacent nodes ringed. It is composed of n-simplex facets, along with all truncated n-simplices.

In four-dimensional Euclidean geometry, the 16-cell honeycomb is one of the three regular space-filling tessellations, represented by Schläfli symbol {3,3,4,3}, and constructed by a 4-dimensional packing of 16-cell facets, three around every face.

In the field of hyperbolic geometry, the hexagonal tiling honeycomb is one of 11 regular paracompact honeycombs in 3-dimensional hyperbolic space. It is paracompact because it has cells composed of an infinite number of faces. Each cell is a hexagonal tiling whose vertices lie on a horosphere, a surface in hyperbolic space that approaches a single ideal point at infinity.

The icosahedral honeycomb is one of four compact regular space-filling tessellations in hyperbolic 3-space. With Schläfli symbol {3,5,3}, there are three icosahedra around each edge, and 12 icosahedra around each vertex, in a regular dodecahedral vertex figure.

In the geometry of hyperbolic 3-space, the order-3-7 hexagonal honeycomb or a regular space-filling tessellation with Schläfli symbol {6,3,7}.

In the geometry of hyperbolic 3-space, the dodecahedral-icosahedral honeycomb is a uniform honeycomb, constructed from dodecahedron, icosahedron, and icosidodecahedron cells, in a rhombicosidodecahedron vertex figure.

In the geometry of hyperbolic 3-space, the order-4 dodecahedral honeycomb is one of four compact regular space-filling tessellations. With Schläfli symbol {5,3,4}, it has four dodecahedra around each edge, and 8 dodecahedra around each vertex in an octahedral arrangement. Its vertices are constructed from 3 orthogonal axes. Its dual is the order-5 cubic honeycomb.

In the field of hyperbolic geometry, the order-4 hexagonal tiling honeycomb arises as one of 11 regular paracompact honeycombs in 3-dimensional hyperbolic space. It is paracompact because it has cells composed of an infinite number of faces. Each cell is a hexagonal tiling whose vertices lie on a horosphere: a flat plane in hyperbolic space that approaches a single ideal point at infinity.

The order-4 octahedral honeycomb is a regular paracompact honeycomb in hyperbolic 3-space. It is paracompact because it has infinite vertex figures, with all vertices as ideal points at infinity. Given by Schläfli symbol {3,4,4}, it has four ideal octahedra around each edge, and infinite octahedra around each vertex in a square tiling vertex figure.

In geometry, the order-4 square hosohedral honeycomb is a regular space-filling tessellation with Schläfli symbol {2,4,4}. It has 4 square hosohedra {2,4} around each edge. In other words, it is a packing of infinitely tall square columns. It is a degenerate honeycomb in Euclidean space, but can be seen as a projection onto the sphere. Its vertex figure, a square tiling is seen on each hemisphere.

In the geometry of hyperbolic 3-space, the order-4 square tiling honeycomb is one of 11 paracompact regular honeycombs. It is paracompact because it has infinite cells and vertex figures, with all vertices as ideal points at infinity. Given by Schläfli symbol {4,4,4}, it has four square tilings around each edge, and infinite square tilings around each vertex in a square tiling vertex figure.

The order-5 cubic honeycomb is one of four compact regular space-filling tessellations in hyperbolic 3-space. With Schläfli symbol {4,3,5}, it has five cubes {4,3} around each edge, and 20 cubes around each vertex. It is dual with the order-4 dodecahedral honeycomb.

The order-5 dodecahedral honeycomb is one of four compact regular space-filling tessellations in hyperbolic 3-space. With Schläfli symbol {5,3,5}, it has five dodecahedral cells around each edge, and each vertex is surrounded by twenty dodecahedra. Its vertex figure is an icosahedron.
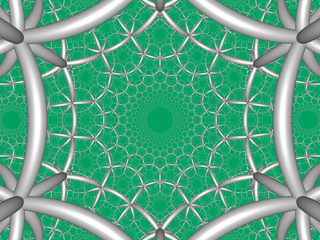
In the field of hyperbolic geometry, the order-5 hexagonal tiling honeycomb arises as one of 11 regular paracompact honeycombs in 3-dimensional hyperbolic space. It is paracompact because it has cells composed of an infinite number of faces. Each cell consists of a hexagonal tiling whose vertices lie on a horosphere, a flat plane in hyperbolic space that approaches a single ideal point at infinity.

The order-6 cubic honeycomb is a paracompact regular space-filling tessellation in hyperbolic 3-space. It is paracompact because it has vertex figures composed of an infinite number of facets, with all vertices as ideal points at infinity. With Schläfli symbol {4,3,6}, the honeycomb has six ideal cubes meeting along each edge. Its vertex figure is an infinite triangular tiling. Its dual is the order-4 hexagonal tiling honeycomb.

The order-6 dodecahedral honeycomb is one of 11 paracompact regular honeycombs in hyperbolic 3-space. It is paracompact because it has vertex figures composed of an infinite number of faces, with all vertices as ideal points at infinity. It has Schläfli symbol {5,3,6}, with six ideal dodecahedral cells surrounding each edge of the honeycomb. Each vertex is ideal, and surrounded by infinitely many dodecahedra. The honeycomb has a triangular tiling vertex figure.
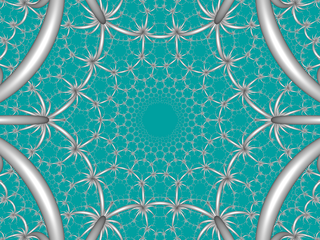
In the field of hyperbolic geometry, the order-6 hexagonal tiling honeycomb is one of 11 regular paracompact honeycombs in 3-dimensional hyperbolic space. It is paracompact because it has cells with an infinite number of faces. Each cell is a hexagonal tiling whose vertices lie on a horosphere: a flat plane in hyperbolic space that approaches a single ideal point at infinity.
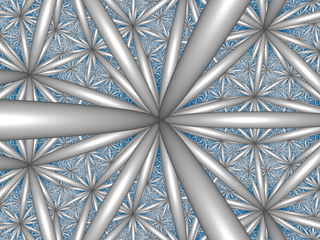
In hyperbolic 3-space, the order-6 tetrahedral honeycomb is a paracompact regular space-filling tessellation. It is paracompact because it has vertex figures composed of an infinite number of faces, and has all vertices as ideal points at infinity. With Schläfli symbol {3,3,6}, the order-6 tetrahedral honeycomb has six ideal tetrahedra around each edge. All vertices are ideal, with infinitely many tetrahedra existing around each vertex in a triangular tiling vertex figure.

The quarter cubic honeycomb, quarter cubic cellulation or bitruncated alternated cubic honeycomb is a space-filling tessellation in Euclidean 3-space. It is composed of tetrahedra and truncated tetrahedra in a ratio of 1:1. It is called "quarter-cubic" because its symmetry unit – the minimal block from which the pattern is developed by reflections – consists of four such units of the cubic honeycomb.

In geometry, the quarter hypercubic honeycomb is a dimensional infinite series of honeycombs, based on the hypercube honeycomb. It is given a Schläfli symbol q{4,3...3,4} or Coxeter symbol qδ4 representing the regular form with three quarters of the vertices removed and containing the symmetry of Coxeter group for n ≥ 5, with = and for quarter n-cubic honeycombs = .
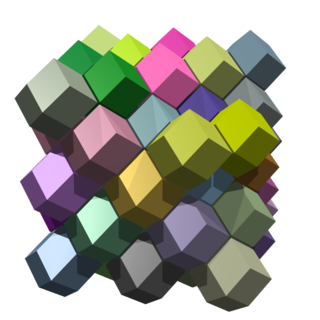
The rhombic dodecahedral honeycomb is a space-filling tessellation in Euclidean 3-space. It is the Voronoi diagram of the face-centered cubic sphere-packing, which has the densest possible packing of equal spheres in ordinary space.

In geometry, the simplectic honeycomb is a dimensional infinite series of honeycombs, based on the affine Coxeter group symmetry. It is given a Schläfli symbol {3[n+1]}, and is represented by a Coxeter-Dynkin diagram as a cyclic graph of n+1 nodes with one node ringed. It is composed of n-simplex facets, along with all rectified n-simplices. It can be thought of as an n-dimensional hypercubic honeycomb that has been subdivided along all hyperplanes , then stretched along its main diagonal until the simplices on the ends of the hypercubes become regular. The vertex figure of an n-simplex honeycomb is an expanded n-simplex.

In the geometry of hyperbolic 3-space, the square tiling honeycomb is one of 11 paracompact regular honeycombs. It is called paracompact because it has infinite cells, whose vertices exist on horospheres and converge to a single ideal point at infinity. Given by Schläfli symbol {4,4,3}, it has three square tilings, {4,4}, around each edge, and six square tilings around each vertex, in a cubic {4,3} vertex figure.
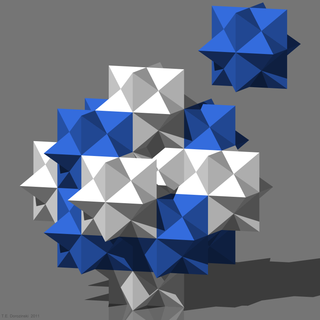
The stellated rhombic dodecahedral honeycomb is a space-filling tessellation in Euclidean 3-space made up of copies of stellated rhombic dodecahedron cells. Six stellated rhombic dodecahedra meet at each vertex. This honeycomb is cell-transitive, edge-transitive and vertex-transitive.

In four-dimensional euclidean geometry, the tesseractic honeycomb is one of the three regular space-filling tessellations, represented by Schläfli symbol {4,3,3,4}, and constructed by a 4-dimensional packing of tesseract facets.

The tetragonal disphenoid tetrahedral honeycomb is a space-filling tessellation in Euclidean 3-space made up of identical tetragonal disphenoidal cells. Cells are face-transitive with 4 identical isosceles triangle faces. John Horton Conway calls it an oblate tetrahedrille or shortened to obtetrahedrille.

The tetrahedral-octahedral honeycomb, alternated cubic honeycomb is a quasiregular space-filling tessellation in Euclidean 3-space. It is composed of alternating regular octahedra and tetrahedra in a ratio of 1:2.

The triakis truncated tetrahedral honeycomb is a space-filling tessellation in Euclidean 3-space made up of triakis truncated tetrahedra. It was discovered in 1914.

The triangular prismatic honeycomb or triangular prismatic cellulation is a space-filling tessellation in Euclidean 3-space. It is composed entirely of triangular prisms.

The triangular tiling honeycomb is one of 11 paracompact regular space-filling tessellations in hyperbolic 3-space. It is called paracompact because it has infinite cells and vertex figures, with all vertices as ideal points at infinity. It has Schläfli symbol {3,6,3}, being composed of triangular tiling cells. Each edge of the honeycomb is surrounded by three cells, and each vertex is ideal with infinitely many cells meeting there. Its vertex figure is a hexagonal tiling.

In hyperbolic geometry, a uniform honeycomb in hyperbolic space is a uniform tessellation of uniform polyhedral cells. In 3-dimensional hyperbolic space there are nine Coxeter group families of compact convex uniform honeycombs, generated as Wythoff constructions, and represented by permutations of rings of the Coxeter diagrams for each family.

In mathematics, a Voronoi diagram is a partition of a plane into regions close to each of a given set of objects. In the simplest case, these objects are just finitely many points in the plane. For each seed there is a corresponding region consisting of all points of the plane closer to that seed than to any other. These regions are called Voronoi cells. The Voronoi diagram of a set of points is dual to its Delaunay triangulation.
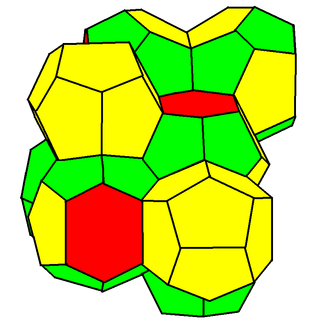
In geometry, the Weaire–Phelan structure is a complex 3-dimensional structure representing an idealised foam of equal-sized bubbles. In 1993, Trinity College Dublin physicist Denis Weaire and his student Robert Phelan found in computer simulations of foam that this structure was a better solution of the "Kelvin problem" than the previous best-known solution, the Kelvin structure.